On-Demand Outsourcing BPO Services for Healthcare Providers With 24/7 Coverage!
Save up to 70% on staffing costs!
Browse Specialty Staffing ServicesSafe, Economical and Effective Edible Algal Vaccines
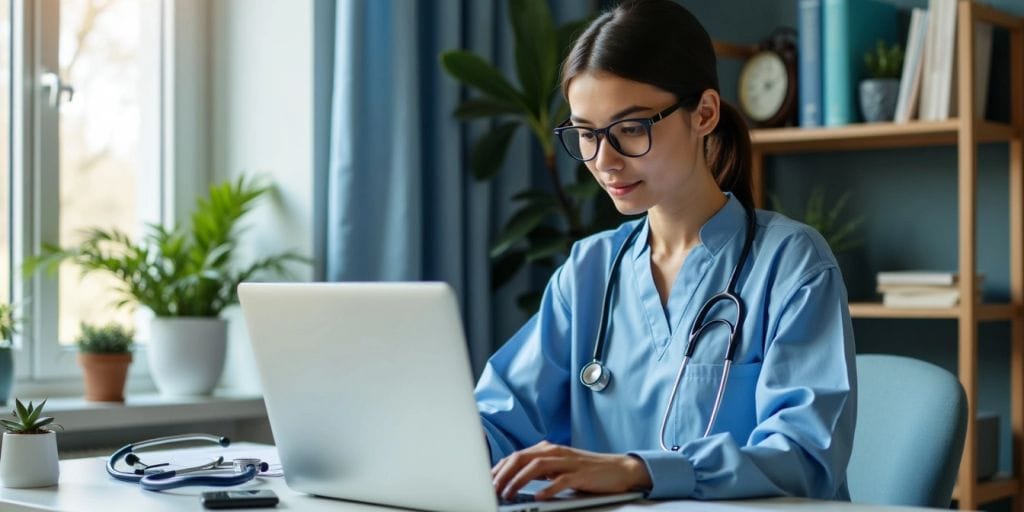
Author: Arrani Ashritha
Department of Pharmacology, G. Pulla Reddy college of pharmacy, Osmania University, Hyderabad, Telangana, India – 500 028.
Abstract
Vaccines are biological preparations that promote immunity and contributed significantly to the 19th-century research. It contains proteins that are similar to virus causing diseases and is usually made up of weakened or killed forms of microbes. They trigger the immune system to recognize and produce the antigens (Kurup& Thomas, 2020). Edible vaccine is a type of vaccine in which selected genes are introduced into plants and the transgenic plant is prompted to produce encoded proteins (Jyoti Saxena& Shweta Rawat, 2013). Edible vaccines are prepared from genetically modified plants. They can be produced by integrating transgene into a selected plant cell. Edible vaccines are currently designed for animal and human use (Kurup &Thomas, 2020). Compared with other common vaccines, edible vaccines are more economical, efficacious, and harmless. They promise a better prevention approach (Maxwell, 2014; Lal et al., 2007; van der Laan et al., 2006).
Edible vaccines provide great scope to reduce various diseases such as measles, hepatitis B, cholera, diarrhea, etc., especially in developing countries (Saxena et al., 2006). Edible vaccines are also known as oral vaccines or food vaccines or dietary vaccines. This article prioritizes the development of oral vaccines and the various ways in which the technology has developed over years.
Keywords: edible vaccines, algal vaccines, peanut allergy, classical swine flu, malaria.
Introduction
A vaccine is a biological preparation whose goal is to stimulate the immune system by stimulating the production of the antibodies. The idea of vaccination was first introduced by Edward Jenner of smallpox in 1796 (Ulmer, Valley and Rappuoli, 2006). Regular vaccines have several restrictions. One of the major problems is the security concern. Another restriction is the need for storage under refrigerated conditions. Vaccines are typically manufactured by industrial processes, thus making them costly and beyond reach in developing countries. For this reason, edible vaccines are seen as ideal substitution for conventional vaccines (Xing Santosuosso et al., 2004; Lycke & Bemark, 2010; Lycke, 2012).
Edible vaccines are usually plants that produce antigens, thus requiring basic agricultural knowledge. The process of purification and downstream processing (DSP) make the conventional vaccines more expensive but these processes are eliminated in edible vaccines making them cost-efficient. The principle of edible vaccines is, to convert dietary foods into potential vaccines to prevent infectious diseases. It involves introducing desirable genes to plants and then recruiting these genetically modified plants to produce encoded proteins. It has also been found to be used in preventing autoimmune diseases, contraception, cancer treatment, etc. Edible vaccines provide an inexpensive, non-invasive, simple, safe, and effective method of vaccine production (Mason et al., 1992).
Edible vaccines can be easily used. The chances of infection with plant pathogens are very small or insignificant as plant pathogens cannot infect humans. Edible vaccines for various diseases such as measles, cholera, foot and mouth disease, as well as hepatitis B, C, and E are produced in plants such as bananas, tobacco, potatoes, etc (Giddings et al., 2000). Edible algae based vaccination is a vaccine strategy under the preliminary research of combining a genetically engineered sub-unit vaccine with an immunological adjuvant in Chlamydomonas reinhardtii microalgae (Specht &Mayfield, 2014). Algal vaccines are similar to plant vaccines. Algae are sometimes called single-cell water-borne plants. Researchers often develop algal vaccines associated with Chlamydomonas reinhardtii, Dunaliella salina, and cyanobacteria (Ma et al., 2020).
History of edible vaccines
Hiat et al. in 1989 tried to produce antibodies in plants that could fulfill the purpose as vaccines, thus initiating research into Edible vaccines. The first report of an edible vaccine (a surface protein from Streptococcus) in tobacco, at 0.02% of the total leaf protein level, emerged in 1990 in the form of a patent application published under an international patent cooperation treaty. In 1992, Arntzen and colleagues introduced the concept of genetically modified plants as a system for the production and delivery of sub unit vaccines using edible tissues of transgenic crop plants. They found that this concept could overcome the limitations of traditional vaccines, thereby initiating the research on edible vaccines (Mor et al., 1998). In the 1990s, Streptococcus mutants surface protein antigen A was expressed for the first time in tobacco. In the same year, the successful expression of hepatitis B surface antigen (HBsAg) in tobacco plants was also achieved (Mason et al., 1992). To prove that plant derived HBsAg could stimulate mucosal immune responses through oral route, potato tubers were used as an expression system and were optimized to enhance accumulation of protein in plant tubers (Richter et al., 2000). Parallel to evaluation of HBsAg extracts from the plant, Mason, and Arntzen examined plant manifestations in other vaccine candidates including labile toxin B subunit (LT-B) enterotoxigenic Escherichia coli (ETEC) and capsid protein of the virus Norwalk. Plant-derived proteins that are properly synthesized into active oligomers may receive the expected immune response when given orally in animals (Mason et al., 1998).
In 1998, a new era in vaccination was launched in which researchers supported by the National Institute of Allergy and Infectious Diseases (NIAID) demonstrated for the first time that an edible vaccine could safely generate important immune responses in humans. In 2003, Sala and a research team reported that proteins produced from these plants trigger the mucosal immune response which was the main target behind the concept of Edible vaccines (Jyoti Saxena &Shweta Rawat, 2013). In 2003, the first documented algal-based vaccine antigen was reported, consisting of an antigen of foot-and-mouth disease associated with subunit B of cholera toxin, which introduced antigen to the mucosal surfaces in mice. The vaccine was grown in C. reinhardtii algae and provided oral immunization in mice, but was hindered by low vaccine antigen expression levels (Specht &Mayfield, 2014).
Mechanism of action
Edible vaccines mainly stimulate mucosal immunity. Edible vaccines are needed to activate the mucosal immune system (MIS). MIS is the first line of defense as this is where human viruses start their infection. The mucosal surfaces are found lining the digestive tract, the respiratory tract, and the urinary tract. There are many ways by which antigen can enter the gut mucosal layer, namely M cells and macrophages. Macrophages are usually activated by interferon-gamma. This activation leads to macrophages delivering different peptides to helper T cells that further produce antibodies (Johansen et al., 1999). M cells are another way by which the antigens are transported to T cells. Antigenic epitopes are then presented on the APC (Antigen Presenting Cells) with the help of helper T cells, which then activate B cells. The activated B cells then migrate to mesenteric lymph nodes where they mature into plasma cells, which then migrate to the mucosal membranes to release immunoglobulin A (IgA). IgA then produces secretory IgA, which is then transported into the lumen. The production of secretory IgA is another complex process since 50% of secretory IgA (sIgA) in the intestinal (gut) lumen is produced by B1 cells in the lamina propria in a T-cell-independent fashion. These sIgAs are polyreactive and usually recognize foreign antigens. In the lumen, sIgA makes the invading pathogen less effective by reacting with certain antigenic epitopes (Walmsley& Arntzen, 2000).
Drawbacks of conventional vaccines
Conventional (common or standard) vaccines have been (and are) the basis of the body’s immune system to fight many diseases. However, a few problems arise when using this type of vaccine.
Some of these problems are:
- SAFETY-Possible modifications to a dangerous type of live vaccine and failure of immunized vaccines are problems (although rare) that can be found when using standard vaccines
- There is a risk of contamination by non-detected viruses or bacteria.
- SECONDARY EFFECTS – Secondary effects of some common vaccines are one of the problems identified. Typically, these secondary effects are produced only at the local level, with inflammation in the inoculation area. Occasionally there is a fever, and very rare ones may have more serious problems, such as allergies or transitory immunosuppression.
- COLD CHAIN-Conventional vaccines need to be refrigerated (+4 to + 6ºC) properly at right temperature during storage and shipping. In some cases, this requirement makes it difficult to maintain the effectiveness of the vaccine, especially in less developed areas.
- Conventional vaccines are given through injections which causes pain.
- Children and adults do not show interest in vaccination due to fear of injection.
- Conventional vaccines have a shorter storage time.
- Conventional vaccines are more expensive compared to oral vaccines.
- They need high quality tools and equipment to produce a vaccine.
- Conventional vaccines require sterile injection conditions
- Conventional vaccines are prepared in a complex way using sophisticated equipment.
Benefits of edible algal vaccines
- Algae can be easily preserved through lyophilization, and two studies of algae-produced vaccines have confirmed that dried algae stored at room temperature for 6 months or even 20 months show a good antigen activity equivalent to freshly harvested algae, although storage at 37 ° did begin to cause loss of activity over time (Gregory et al., 2013).
- The wall of algal cells appears to be sufficient to withstand severe conditions within the abdomen, as very small antigen depletion was observed after all cells were exposed to pepsin at pH 1.7 (Dreesen, Hamri &Fussenegger, 2010).
- Algae is an ideal host to transport vaccines without the provision of cold-chain supply, and that cells provide adequate protection for antigens on the way to intestinal lymph tissue, avoiding the additional costs associated with encapsulation.
- Microalgae are very easy to mutate, thus showing higher levels of exposure of foreign genes (Georgopoulou et al., 1988).
- Algae vaccines are cheaper compared to those produced by plants.
- An alga is a food source for many species that include humans (Ellis, 1988).
- Microalgae are resistant to animal pathogens, thus making them an excellent way to produce vaccines
- Compared with other traditional vaccines, edible vaccines promise better protection against disease if properly developed. This is a less expensive, effective and safe model as a vaccine (van der Laan et al., 2006).
- Compared with traditional vaccines, oral vaccines do not require sophisticated equipment for vaccine production.
- Edible vaccines are safer than conventional vaccines and do not require sterile injectable conditions and storage conditions etc. Edible vaccines stimulate both systemic and mucosal responses.
- An edible vaccine can produce complex multimeric proteins that can be produced by the microbial system and are a safe and effective method of vaccine (Kurup &Thomas, 2020).
- They can be produced in bulk. Hence, they are economical
- They can be administered by eating the plant / part of the plant. Therefore, Processing and cleaning steps can be eliminated
- It can be stored at room temperature, unlike standard vaccines that require extensive cold storage conditions.
- The process of transport and distribution can be eliminated if a local / indigenous crop is engineered to produce a vaccine (Hirlekar& Bhairy, 2017).
- Cross-contamination of adjacent yields will not be possible for green algae as they can be grown by confined or encased bioreactors.
- Studies to date show that algae such as Chlamydomonas can produce complex antigens that can stimulate the immune system and are suitable for development as vaccines (Ekam et al., 2006).
- Microalgae thrive on simple cultivation media, does not require complex infrastructure and does not compete with locally grown crops for arable lands.
- Microalgae work much better than taller plants to convert sunlight into biomass.
- As in plants, recombinant antigen found in collected algae biomass does not need to be extracted or purified, as it can dry out and the algal cell wall protects the antigens with a long useful life of up to 20 months without losing efficiency at room temperature. This is especially useful to the developing countries, which often have problems with cold chains in the storage / transportation of conventional vaccines.
- From a technical point of view, algae are preferable as the development of a new algal strain takes about one month, while the establishment of genetically modified plants can take up to one year (Georgopoulou et al., 1988; Ellis, 1988; van der Laan et al., 2006; Kurup &Thomas, 2020).
Limitations
- Sometimes transgene causes allergies. Oral vaccines made from plants may cause allergic reactions during post-transformation, and oral tolerance when administered with oral adjutants in particular, to activate the mucosal immune system, may cause allergic reactions to other proteins contained in the daily diet.
- Repeated delivery of plant-based vaccines may increase T-cell-regulation in contrary to vaccine antigen thereby causing stimulation of hypersensitivity reactions in case of pollen allergy or food allergy (Maxwell, 2014).
- Sometimes DNA (Deoxyribonucleic acid) or antigen may release into water sources through contact with insects or birds with plants that cause water pollution.
- Medicines can accidentally enter the human food chain and affect the population of wildlife (Hirlekar& Bhairy, 2017).
- Selection of plants with stable antigen production can be a difficult, time-consuming, and costly task (Twyman et al., 2005).
- Lack of knowledge about plant biotechnology leading to negative public perceptions, stricter rules, and debates about intellectual property, hinders investment in the pharmaceutical business in Edible vaccines.
- The likelihood of hypersensitive reactions, improved oral tolerance to vaccines, and difficulty in standard dose management are additional limitations (Jacob et al., 2013).
- A major impact on algal cultivation resides in a high risk of cultural contamination by competing pests, which occur both in closed photobioreactors and in high-speed open systems. This issue makes algal biotechnology less expensive but it takes higher administrative costs to prevent that.
- Most of the natural algal species (wild species) used in genetic engineering do not have the necessary genes for the production of nitrogen (nitrate reductase), which makes them more dependent on the nitrogen source provided in the cultivation medium, thus making their survival in the wild highly impossible (Kurup &Thomas, 2020).
Overcomes
- Plant-derived vaccines should be clinically tested under United States investigational new drug application, and also must follow all the regulatory and GMP (Good Manufacturing Practices) requirements (Kim et al., 2004).
- It is necessary to make awareness in the society of the use and benefits of edible vaccines (Zapanta &Ghorab, 2014).
- Checking the stability of the genetically modified plants, algae, and their proper isolation is essential (Kim et al., 2016).
- Prevention of environmental contamination and prevention of serious side effects is mandatory (Lal et al., 2007).
- Growing plants for edible vaccine production requires close monitoring. Transgenic plants require the separation of the greenhouse and the different bodies to ensure that such plants do not release antigenic proteins into the environment in any way (Gunasekaran& Gothandam, 2020).
Use of edible algal vaccines for various diseases
- Classical swine flu virus
Classical swine flu virus (CSFV) is an infectious virus that causes swine flu (Moennig, 2000). Even though vaccines are the leading prevention method against CSFV, attenuated vaccines, and C-strain vaccines have been reported to have lost their ability to differentiate between infected and vaccinated animals (Markowska-Daniel et al., 2001). The protein E2 has great antigenic properties and makes the immune system unique. In research carried out by He et al this E2 protein from the CSFV was expressed in Chlamydomonas reinhardtii (He et al., 2007).Immune experiments were performed on animal models to determine the immunogenicity of the expressed protein. There has been an increase in serum antibody against CSFV when the extract is administered subcutaneously (He et al., 2007).
- Foot and mouth disease
Foot and mouth virus (FMPV) is a major livestock disease and has been largely controlled by vaccination (Sobrino et al., 2001). Both inactivated and attenuated vaccines are used but are generally not considered completely safe. The protein of the Foot and Mouth Disease Virus (FMDV), Virion Protein1 (VP1), contains important epitopes that can produce antibodies (Brown et al., 1991). Cholera Toxin B subunits were used as they are very effective at acting as a mucosal adjuvant that can bind to the intestinal epithelial area using monosialotetrahexosyl ganglioside (GM1) ganglioside receptors. Plasmid pACTBVP1 was transformed using biolistic bombardment into the microalgae Chlamydomonas reinhardtii. After transformation, it was placed under dim light until the cells turned yellow as reported by Suzuki et al.(Suzuki and Bauer, 1992)Selected transformants (streptomycin resistance) were analyzed by Polymerase Chain Reaction (PCR) with ChIL primers. PCR products were then analyzed by Southern blotting. The presence of the combined protein (CTBVP1) was assessed by western blotting. Enzyme Linked Immunosorbent Assay (ELISA) was carried out for quantitative analysis. The combined protein has shown a weak but important affinity for GM1 ganglioside. The research by Sun et al showed that Chlamydomonas expressed CTBVP1 in large numbers (Sun et al., 2003). It also showed that this fusion protein was bound to GM1 ganglioside, which means it can be used as a potential source of mucosal vaccine(Sun et al., 2003).
- Hepatitis B
Hepatitis B is one of the most common chronic illnesses affecting around 350 million people worldwide (Lavanchy, 2004). Hepatitis B surface antigen (HBsAg) has been used as a vaccine for quite some time. HBsAg is often isolated from high-risk patients. Currently, a hepatitis vaccine is produced mainly in yeast (Valenzuela et al., 1982). The HBsAg antibody was expressed in an algal expression vector, Phaeodactylum tricornutum(Hempel et al., 2011).The results of the study showed that the human antibody CL4mAb was expressed and synthesized in the endoplasmic reticulum of microalgae. When the same antibody was introduced to the plant Nicotina tobacum, it showed much lower expression levels (Yano et al., 2004). Protein degradation, which is reported to be a major problem in plants, was not found when the same protein was expressed in P. tricornutum (Muynck et al., 2009). ELISA tests performed on whole proteins and refined proteins showed that this virus binds to the antigen HBsAg with great efficiency. In addition to producing these antibodies, the HBsAg antigen was expressed in P. tricornutum (Hempel et al., 2011). HBsAg is widely used as a hepatitis B vaccine. When exposed to microalgae, 0.7% of the total soluble protein was HBsAg. This antigen was identified by an algae-produced antibody and a commercially produced antibody. In another study, Geng et al. showed genetic modification of HBsAg in the algae Dunaliellasalina. This is done by electroporation (Geng et al., 2003). Chloramphenicol-resistant strains were selected and tested by molecular analysis. The successful integration of HBsAg genes into the Dunaliella salina genome was confirmed by PCR and southern blotting. By doing the ELISA it was found that a large amount of HBsAg protein is expressed by D. salina. This HBsAg has been found to have an immune activity (Chen et al., 2001).
- Human papilloma virus(HPV)
Human papilloma virus accounts for about 6.1% of all cancer cases worldwide. Of those, 99.7% are agents responsible for cervical cancer. More than half of cases are caused by HPV16 (jan m. m. walboomers1 et al.,1999) Ordinary drugs do not work against cervical cancer tumors, they are usually toxic, and can lead to recurrence (possibly 10-20%) (Chen et al., 2001). Hr-HPV-E7 oncoprotein, which is involved in the conversion of harmful cells, is a perfect candidate for the development of vaccines (McLaughlin-Drubin & Münger, 2009). In a study by Demurtas et al. HPV-E7 protein in its attenuated form was expressed in microalgae C. reinhardtii. It has shown positive results in pre-clinical animal models (Demurtas et al., 2013). This antigen has so far been analyzed for biochemical and physiological studies, but expression in algae has now opened up new possibilities. Future activities could detect the excess exposure of this antigen to algae, so that it can be used directly as an HPV vaccine (Alonso et al., 2002).
- Malaria
Malaria is a disease caused by parasitic protozoa Plasmodium falciparum. It is transmitted by the bite of a mosquito. Each year, some 100 million people die, at least 300 to 500 million get infected (Snow et al., 2005). The most advanced and most recent vaccine used against malaria is directly related to sporozoite. This policy is designated by RTS, S / ASO2A. In a study by Dauvillée et al., High levels of granule bound starch synthase (GBSS) bound to starch, which fuses to three malaria vaccines and were then expressed in microalgae C. reinhardtii .It was shown that the amount of starch-antigen accumulated in chloroplast of algae was sufficient to prevent the lethal dose of Plasmodium falciparum in mice. This inhibition was observed due to the inhibition of erythrocyte invasion. In this study, C. reinhardtii is used as a starch in its chloroplast, which makes the vaccine long lasting. Also, this algae has a Generally Regarded as Safe (GRAS) form and is very easy to propagate and cultivate (Dauvillée et al., 2010). In a study conducted by Gregory et al., (2012), Vaccines for the malarial subunit pfs25 and pfs28 were expressed in C. reinhardtii. Both of these units are part of the structure of a vaccine prepared to prevent the transmission of malaria. The algae produced pfs25 and pfs28 were found to have similar structural to native pfs25 and pfs28. This makes the algal expression system the only system for expressing these 2 proteins in a glycosylated form. Structural similarity was identified using monoclonal antibodies that bind only to the right pfs25 and pfs28 (Gregory et al., 2012). In the yeast homologs of pfs25, disulfide bonds were found to be absent, but the algal expression system expressed pfs25 with disulfide bonds (Saxena et al., 2006). It was shown that a-pfs25 but not pfs28 showed significant ability to prevent transmission, consistent with previous works (Gozar et al., 1998; Gozar et al., 2001).
- Staphylococcus aureus
aureus is a Gram-positive bacterium. It belongs to the group of bacteria called Firmicutes. S. aureus is a human pathogen that infects the nasal mucosa and the skin (Lowy, 1998).It is responsible for the bacteremia, which is the cause of secondary infections such as endocarditis, pneumonia, meningitis, etc (Moreillon& Que, 2004). Dreesen et al (2010) reported that the fibronectin-binding protein produced by S. aureus is very important in its management, and is associated with cholera toxin B (Kurup& Thomas, 2020). The protein that binds to fibronectin attaches itself to the extracellular matrix of the host cell (Patti& Höök, 1994). The Cholera Toxin B(CTB) has improved antigen-specific immune response(Sun et al., 1994). CTB-D2 fusion antigen was codon-optimized and exposed to the chloroplast of microalgae C. reinhardtii. CTB-D2 antigen was resistant to conditions that mimic the abdominal environment and low pH. It also binds to GM1 ganglioside and triggers a systemic and mucosal immune response. CTB-D2 antigen-expressing algae was strengthened and given to mice, which were protected from the deadly doses of Staphylococcus aureus (Sun et al., 1994).
- Covid-19
The novel coronavirus (2019-nCoV), more commonly identified as the coronavirus disease 2019 (COVID-19), named officially by the World Health Organization (WHO) on the 12th of January 2020, had its first outbreak in the Huanan South China Seafood Market, located in Wuhan City, Hubei Province, China(Guo et al., 2020). According to Sami et al (2020) algae can be recommended as a treatment toSARS-Cov-2(Severe Acute Respiratory Syndrome Coronavirus 2), both preventive and curative (Sami et al., 2020). It was suggested that sulfated polysaccharides can inhibit viral infection by interfering with S-protein of SARS-CoV-2 that binds to heparin sulfate co-receptor in host tissues. Kwon et al reported in vitro effects of strong binding between certain sulfated polysaccharides and S-protein. The use of these non-anticoagulant polysaccharides can be oral delivery, a moderate dose inhaler or nasal spray, as heparin is not available orally but fucoidans extracted from edible sea polysaccharides are categorized as safe GRAS (Kwon et al., 2020). C-phycocyanin, a pigment-binding protein found in Spirulina blue-green algae, enhances anti-tumor, anti-inflammation, and anti-oxidant activities (Cian et al., 2012).
Tzachor et al (2021) used aqueous extracts of Spirulina as a therapy for cytokine storm and reported a reduction of tumor necrosis factor (TNF)-α secretion levels induced by macrophage and monocyte (Tzachor et al., 2021). Anti-TNF therapy or TNF-α blockers are important to reduce inflammation-driven capillary leak caused by the key inflammatory cytokines which deteriorate the lung function of COVID-19 patients (Robinson et al., 2020). Algal nutraceuticals with their anti-inflammatory, antimicrobial, immunostimulatory, and immunomodulatory properties are also important for boosting immunity, preventing diseases, and treating disorders associated with severe SARS-CoV-2 infections, such as anti-inflammatory treatment and tissue repair (Ratha et al., 2020). C-phycoyanin, a pigment-binding protein, with the properties of anti-inflammation, anti-oxidant, and anti-tumor was tested to reduce the secretion levels of a protein, which causes cytokine storms in COVID-19 patients (Tzachor et al., 2021). Although it will not replace SARS-CoV-2 vaccinations, the algae extract may be used as a dietary supplement to prevent cytokine storms once the patients are diagnosed, especially those high-risk populations including the elderly and those with severe medical conditions this is because the influx of those pro-inflammatory cytokines such as TNF-α, interleukin (IL)-2, IL-7, IL-10, macrophage inflammatory protein-1A (MIP-1A), and monocyte chemo attractant protein-1 (MCP-1) found in critically ill COVID–19 patients may cause acute respiratory distress syndrome (ARDS), which is the main cause of their death (Ruan et al., 2020; Mcgonagle et al., 2020; Berndt, et al., 2021).
The current ongoing researches use single-celled alga C. reinhardtii where one research group in Italy, research is being carried out by the Laboratory of Photosynthesis and Bioenergy of the Department of Biotechnology at the University of Verona, directed by professors Roberto Bassi and Luca Dall’Osto they introduced a DNA sequence corresponding to the SARS-CoV-2 RBD (Receptor Binding Domain) protein by adopting nuclear transgenesis and chloroplast transformation ability to perform genetic engineering, especially on the single-celled alga of the model organism Chlamydomonas reinhardtii, has provided the basis for contributing to the development of an oral vaccine against the recently emerged SARS-COV-2 viral strain responsible for the current pandemic threatening the global health. One of the great advantages of algae is that they grow and multiply quite quickly. According to Cutolo, if contamination is prevented, it is possible to accumulate up to 1 mg of the recombinant antigen for each gram of biomass of dried algae. Additionally, Berndt et al. 2021 utilized C. reinhardtii to produce recombinant SARS-CoV-2 spike RBD protein and found out that it has a similar affinity as mammalian expressed in specifically binding to the recombinant Angiotensin Converting Enzyme 2 (ACE2) protein, showing the potential of using algae to produce functional and correctly-folded recombinant spike RBD protein which could be utilized in large-scale serological tests or as potential vaccine antigens (Berndt et al., 2021).
A Biotech Company in Israel, Trans Algae identified and inserted a portion of the SARS-COV2 spike protein into the algae for the manufacturing of spike protein to stimulate immune responses, while claiming that adding the spike protein in tiny amounts does not change the safety profile of the algae for humans. It’s very likely that they are using the same algae model C. reinhardtii for genetic modification and accumulate large amounts of the antigen—modified algae that would be lyophilized to generate an oral capsule (Sami et al., 2020). The key feature of using algae for vaccine production is that an oral vaccine can be produced by lyophilizing and encapsulating the algae, where their cell wall could protect the antigens and bioactive molecule from the harsh gastric environment, ensuring its arrival to the intestinal immune system (Gunasekaran &Gothandam, 2020).
- Peanut allergy
Peanut allergy is an adverse reaction of IgE (Immunoglobulin E) to a set of proteins found in the legume Arachis hypogaea (peanuts). Patients who are allergic to peanuts show a complete T-Helper type 2 (TH2)-polarized response to peanuts and IgE that detects one or more allergens (Flinterman et al., 2008). After exposure to peanuts, IgE in mast cells that live in tissues and circulating basophils bind to the cognate allergen causing rapid deterioration and release of histamine and inflammatory molecules. This flow leads to allergic reactions ranging from minor rashes and intestinal depression to fatal systemic anaphylaxis and organ failure (Hsu & MacGlashan, 1996).
Algal produced Ara h1 (Arachis Hypogaea-1 antigen) core domain and Ara h2 (Arachis Hypogaea-2 antigen) have reduced affinity for IgE from peanut-allergy patients. It was also found that immunotherapy using an Ara h 1 base derived from algae provides protection from peanut induced anaphylaxis in the murine model of peanut allergy. The microalgae used to produce the vaccine for peanut allergy is Chlamydomonas reinhardtii (Gregory et al., 2016).
- Hypertension
Hypertension, also known as raised or high blood pressure, is a condition in which the blood vessels have persistently raised pressure. Unlike traditional hypertension therapies, immunotherapies act as a promising alternative as they are cheaper and offer better patient compliance. A chimeric antigen intended to prevent hypertension, consisting of a genetic fusion between Angiotensin II and a Hepatitis B antigen (HBcAg) serving as a carrier, was the first algal vaccine to be expressed from nuclear genome without chloroplast targeting(Specht &Mayfield, 2014). This candidate immunogen designated as HBcAgII has been expressed in algal specie Chlamydomonas reinhardtii, which serves as an excellent vaccine expression system and delivery host. Transgenic C. reinhardtii lines have been improved, and the expected recombinant protein has been detected by Western blot and ELISA analysis. The expression levels of this recombinant protein in some transgenic lines have reached even upto 0.05% of the total soluble protein which serves as a great advancement in creating edible algal vaccines for treatment of hypertension (Soria-Guerra et al., 2014).
- White spot syndrome
White spot disease (WSD) is a dangerous viral disease of various shrimp species that has caused high mortality for decades. This disease is caused by White Spot Syndrome Virus (WSSV). White spot disease is leading to huge losses in the shrimp industry worldwide. However, the mechanism of entry and transmission of the virus into the shrimp cells is unknown. A binding in vitro test showed envelope protein viral protein 28 (VP28) combined with enhanced green fluorescence protein (VP28-EGFP) binding to shrimp cells. This provides direct evidence that VP28-EGFP can bind to shrimp cells at pH 6.0 within 0.5 hours. However, proteins were observed to enter the cytoplasm 3hours post-adsorption. Meanwhile, plaque inhibition tests showed that a polyclonal antibody against VP28 (a large WSSV envelope protein) could weaken WSSV and prevent viral infection. The outcome of the ELISA test also confirmed that the protein envelope VP28 could compete with WSSV to bind to shrimp cells. Overall, VP28 of the WSSV can bind to shrimp cells as an attached protein and can help the virus enter the cytoplasm. During white spot syndrome (WSSV) infection, the interaction between viral enzyme proteins and cell surface protein receptors on the target cells is an important step in viral entry and replication (Kiataramgul et al., 2020).
An oral WSSV shrimp control program has been developed based on engineered edible microalgae. In this case, the codon-optimized synthetic gene of WSSV VP28 was incorporated into the chloroplast genome C. reinhardtii. Oral administration of transgenic algae increased the survival rate of the shrimps exposed to WSSV compared to the control group. Algae lacking a cell wall can last for at least 80 minutes under conditions similar to shrimp digestion system. In WSSV infection tests, a high survival rate (87%) was recorded in shrimp fed with codon-optimized VP28 line mixed with their diet, indicating that this line can be used in control WSSV spread in shrimp communities. This algal strategy provides a new, effective, fast, and cost-effective way to control other diseases in aquatic animals through oral delivery. (Kiataramgul et al., 2020).
Table-1, Available Edible Algal Vaccines for Various diseases
S.no | Diseases | Host microalgae- |
1, | Foot and mouth disease | ChlamydomonasReinhardtii |
2, | Classical swine flu | ChlamydomonasReinhardtii |
3, | Human Papilloma Virus | ChlamydomonasReinhardtii |
4, | Staphylococcus aureus | ChlamydomonasReinhardtii |
5, | Hepatitis B | Phaeodactylumtricornutum, Dunaliellasalina |
6, | Malaria | ChlamydomonasReinhardtii |
7, | White spot syndrome | ChlamydomonasReinhardtii |
8, | Hypertension (angiotensin ii) | ChlamydomonasReinhardtii |
9, | Covid-19 | ChlamydomonasReinhardtii
|
10, | Peanut allergy | ChlamydomonasReinhardtii |
Conclusion
Edible vaccines hold great promises as a cost-effective, easy-to-administer, easy-to-store vaccine, especially for the poor and developing countries. Initially believed to be useful only for preventing infectious diseases, it has also found application in the prevention of autoimmune diseases, birth control, cancer therapy, and etc. There is growing acceptance of genetically-engineered crops in both industrial and developing nations. So in the future, there is a lot of scope for the introduction of edible vaccines as the primary method of vaccination.
Among the edible vaccines, edible algal vaccines are more prominent to become the major method of vaccination. Because, from the research available to date, it is clear that algae like Chlamydomonas can produce complex vaccine antigens that can produce immunogenic responses that are suited for their target roles as vaccines. Algae are very important for rapidly testing many versions of potential chimeric vaccine molecules. An algal-produced human vaccine production platform will likely become an alternative for very expensive vaccines like HPV, or for novel vaccines against diseases for which there is no alternative yet. The costs and considerations of the order of storage, delivery, and administration in limited resource settings suggests that plant or algal production may be the only possible alternative for largescale less expensive vaccines. Thus, this area requires increased attention from research funding agencies and also investment from the pharmaceutical industry to get Edible algal vaccines to public use as early as possible.
Acknowledgment
The author is grateful to Mr. Mohammed Abdul Samad, Research Scholar, Department of Pharmacology, G. Pulla Reddy College of Pharmacy, Osmania University, Hyderabad, Telangana, India for his immense support in guiding throughout the review work.
Conflict of interest
The authors declared no conflict of interest.
References
Alonso, L. G., Garcia-Alai, M. M., Nadra, A. D., Lapena, A. N., Almeida, F. L., Gualfetti, P., and Prat-Gay, G. D. 2002. High-risk (HPV16) human papillomavirus E7 oncoprotein is highly stable and extended, with conformational transitions that could explain its multiple cellular binding partners. Biochemistry, 41(33):10510–10518.
Berndt, A., Smalley, T., Ren, B., Badary, A., Sproles, A., Fields, F., Torres-Tiji, Y., Heredia, V., Mayfield, S. 2021. Recombinant production of a functional SARS-CoV-2 spike receptor binding domain in 4 the green algae Chlamydomonas reinhardtii’. PLoS ONE, 16(11):e0257089.
Brown, L. E., Sprecher, S. L. and Keller, L. R. 1991. Introduction of exogenous DNA into Chlamydomonas reinhardtii by electroporation. Molecular and Cellular Biology, 11(4):2328–2332.
Chen, Y., Wang, Y., Sun, Y. et al. 2001. Highly efficient expression of rabbit neutrophil peptide-1 gene in Chlorella ellipsoidea cells. Current Genetics, 39(5–6):365–370.
Cian RE, López-Posadas R, Drago SR, de Medina FS, Martínez-Augustin O. 2012. Immunomodulatory properties of the protein fraction from Phorphyra columbina. J Agric Food Chem, 60(33):8146-54.
Dauvillée D, Delhaye S, Gruyer S, Slomianny C, Moretz SE, d’Hulst C, et al. 2010. Engineering the Chloroplast Targeted Malarial Vaccine Antigens in Chlamydomonas Starch Granules. PLoS ONE, 5(12):e15424.
Demurtas OC, Massa S, Ferrante P, Venuti A, Franconi R, Giuliano G. 2013. A Chlamydomonas-Derived Human Papillomavirus 16 E7 Vaccine Induces Specific Tumor Protection. PLoS ONE, 8(4): e61473.
Dreesen IA, Charpin-El Hamri G, Fussenegger M. 2010. Heat-stable oral alga-based vaccine protects mice from Staphylococcus aureus infection. J Biotechnology, 145(3):273-80.
Ekam VS, Udosen EO, Chigbu AE. 2006. Comparative effect of carotenoid complex from Golden Neo-Life Dynamite (GNLD) and carrot extracted carotenoids on immune parameters in albino Wistar rats. Niger J Physiol Sci, 21(1-2):1-4.
Ellis, A. E. (1988) Current aspects of fish vaccination. Diseases of aquatic organisms, 4(2):159-164.
Flinterman AE, Knol EF, Lencer DA, Bardina L, den Hartog Jager CF, Lin J, et al. 2008. Peanut epitopes for IgE and IgG4 in peanut-sensitized children in relation to severity of peanut allergy. J Allergy Clin Immunol, 121(3):737-743.e10.
Geng, D., Wang, Y., Wang, P. et al. 2003. Stable expression of hepatitis B surface antigen gene in Dunaliella salina (Chlorophyta). Journal of Applied Phycology, 15:451–456.
Georgopoulou, U., Dabrowski, K., Sire, M.F. et al. 1988. Absorption of intact proteins by the intestinal epithelium of trout, Salmo gairdneri. Cell Tissue Res, 251:145–152.
Giddings, G., Allison, G., Brooks, D. et al. 2000. Transgenic plants as factories for biopharmaceuticals. Nat Biotechnol, 18(11):1151–1155.
Gozar MM, Muratova O, Keister DB, Kensil CR, Price VL, Kaslow DC. 2001. Plasmodium falciparum:immunogenicity of alum-adsorbed clinical-grade TBV25-28, a yeast-secreted malaria transmission-blocking vaccine candidate. Exp Parasitol, 97(2):61–69.
Gozar, M. M. G., Price, V. L. and Kaslow, D. C. 1998. Saccharomyces cerevisiae-secreted fusion proteins Pfs25 and Pfs28 elicit potent Plasmodium falciparum transmission-blocking antibodies in mice. Infection and Immunity, 66(1): 59–64.
Gregory JA, Li F, Tomosada LM, Cox CJ, Topol AB, Vinetz JM, et al. 2012. Algae-Produced Pfs25 Elicits Antibodies That Inhibit Malaria Transmission. PLoS ONE, 7(5): e37179.
Gregory, J. A, Topol, A. B, Doerner, D. Z, and Mayfield, S. 2013. Alga-produced cholera toxin-pfs25 fusion proteins as oral vaccines. Applied and Environmental Microbiology, 79(13):3917–3925.
Hsu, C. and MacGlashan, D. 1996. ‘IgE antibody up-regulates high affinity IgE binding on murine bone marrow-derived mast cells’, Immunology Letters, 52(2–3):129–134.
Gregory, J.A, Shepley-McTaggart, A, Umpierrez, M, Hurlburt, B.K, Maleki, S.J, Sampson, H.A, Mayfield, S.P, Berin, M.C. 2016. Immunotherapy using algal-produced Ara h 1 core domain suppresses peanut allergy in mice. Plant Biotechnology Journal, 14(7):1541–1550.
- Gunasekaran, K.M. Gothandam. 2020. A review on edible vaccines and their prospects. Brazilian Journal of Medical and Biological Research, 53:e8749.
Guo, YR, Cao, QD, Hong, ZS. et al. 2020. The origin, transmission and clinical therapies on coronavirus disease 2019 (COVID-19) outbreak – an update on the status. Military Med Res, 7(1):11.
D.M. He, K.X. Qian, G.F. Shen, Z.F. Zhang, L.I. Y.N, Z.L. Su, H.B. Shao. 2007. Recombination and expression of classical swine fever virus (CSFV) structural protein E2 gene in Chlamydomonas reinhardtii chroloplasts, Colloids Surfaces B Biointerfaces, 55(1):26–30.
Hempel, F.; Lau, J.; Klingl, A.; Maier, U.G. 2011. Algae as Protein Factories: Expression of a Human Antibody and the Respective Antigen in the Diatom Phaeodactylum tricornutum. PLoS ONE, 6(12): e28424.
Hirlekar R. and Bhairy S. 2017. Edible vaccines: an advancement in oral immunization. Asian Journal of Pharmaceutical and Clinical Research, 10 (2): 82-88.
- S. Jacob, S. Cherian, T. G. Sumithra, O. K. Raina, and M. Sankar. 2013. Edible vaccines against veterinary parasitic diseases—Current status and future prospects. Vaccine, 31(15):1879–1885.
Johansen F-E, Pekna M, Norderhaug IN et al. 1999. Absence of epithelial immunoglobulin A transport, with increased mucosal leakiness, in polymeric immunoglobulin receptor/secretory component-deficient mice. Journal of Experimental Medicine, 190(7):915-921.
Saxena J., Rawat S. (2014) Edible Vaccines. In: Ravi I., Baunthiyal M., Saxena J. (eds) Advances in Biotechnology. Springer, New Delhi, 207-226.
Kiataramgul, A, Maneenin, S, Purton, S, Areechon, N, Hirono, I, Brocklehurst, T.W, Unajak, S. 2020. An oral delivery system for controlling white spot syndrome virus infection in shrimp using transgenic microalgae. Aquaculture, 521:735022
Kim N-S, Mbongue JC, Nicholas DA, Esebanmen GE, Unternaehrer JJ, Firek AF, et al. 2016. Chimeric Vaccine Stimulation of Human Dendritic Cell Indoleamine 2, 3-Dioxygenase Occurs via the Non-Canonical NF-κB Pathway. PLoS ONE, 11(2):e0147509.
Kim, TG. , Galloway, D.R. & Langridge, W.H.R. 2004. Synthesis and assembly of anthrax lethal factor-cholera toxin B-subunit fusion protein in transgenic potato. Mol Biotechnol, 28(3):175–183.
Kurup, V.M, Thomas, J. 2020. Edible Vaccines: Promises and Challenges. Molecular Biotechnology, 62(2):79–90.
Kwon, P.S., Oh, H., Kwon, SJ. et al. 2020. Sulfated polysaccharides effectively inhibit SARS-CoV-2 in vitro. Cell Discovery, 6(1):1-4.
Van der Laan JW, Minor P, Mahoney R, Arntzen C, Shin J, Wood D. 2006. WHO informal consultation on scientific basis for regulatory evaluation of candidate human vaccines from plants. Vaccine, 24(20):4271–4278.
Lal, P. et al. 2007. EDIBLE VACCINES: CURRENT STATUS AND FUTURE. Indian Journal of Medical Microbiology, 25(2):93–102.
Lavanchy D. 2004. Hepatitis B virus epidemiology, disease burden, treatment, and current and emerging prevention and control measures. Journal of Viral Hepatitis, 11(2):97–107.
Lowy, F. D. 1998. Staphylococcus aureus Infections. New England Journal of Medicine, 339(8):520–532.
Lycke, N. 2012. Recent progress in mucosal vaccine development: potential and limitations. Nature Reviews Immunology, 12(8):592–605.
Lycke, N., Bemark, M. 2010. Mucosal adjuvants and long-term memory development with special focus on CTA1-DD and other ADP-ribosylating toxins. Mucosal Immunology, 3(6); 556–566.
Ma K, Bao Q, Wu Y, Chen S, Zhao S, Wu H and Fan J. 2020. Evaluation of Microalgae as Immunostimulants and Recombinant Vaccines for Diseases Prevention and Control in Aquaculture. Frontiers in Bioengineering Biotechnology, 8:1331
Markowska-Daniel I, Collins RA, Pejsak Z. 2001. Evaluation of genetic vaccine against classical swine fever. Vaccine, 19(17-19):2480-2484.
Mason HS, Haq TA, Clements JD, Arntzen CJ. 1998. Edible vaccine protects mice against Escherichia coli heat-labile enterotoxin (LT): potatoes expressing a synthetic LT-B gene. Vaccine, 16(13):1336-43.
Mason, H. S., Lam, D. M., & Arntzen, C. J. 1992. Expression of hepatitis B surface antigen in transgenic plants. Proceedings of the National Academy of Sciences of the United States of America, 89(24):1745–11749.
Maxwell, S. 2014. Analysis of Laws Governing Combination Products, Transgenic Food, Pharmaceutical Products and their Applicability to Edible Vaccines, Brigham Young University Prelaw Review, 28(1):8.
McGonagle D, Sharif K, O’Regan A, Bridgewood C. 2020. The Role of Cytokines including Interleukin-6 in COVID-19 induced Pneumonia and Macrophage Activation Syndrome-Like Disease. Autoimmunity Reviews, 19(6):102537.
McLaughlin-Drubin ME, Münger K. 2009. The human papillomavirus E7 oncoprotein. Virology, 384(2):335-44.
Moennig V. 2000. Introduction to classical swine fever: virus, disease and control policy. Veterinary Microbiology, 73(2-3):93-102.
Mor TS, Gómez-Lim MA, Palmer KE. 1998. Perspective: edible vaccines–a concept coming of age. Trends in Microbiology, 6(11):449-53.
Moreillon P, Que YA. 2004. Infective endocarditis. Lancet, 363(9403):139-49.
De Muynck B, Navarre C, Nizet Y, Stadlmann J, Boutry M. 2009. Different subcellular localization and glycosylation for a functional antibody expressed in Nicotiana tabacum plants and suspension cells. Transgenic Research, 18(3):467-482.
Patti JM, Höök M. 1994. Microbial adhesins recognizing extracellular matrix macromolecules. Current Opinion Cell Biology, 6(5):752-758.
Ratha SK, Renuka N, Rawat I, Bux F. 2020. Prospective options of algae-derived nutraceuticals as supplements to combat COVID-19 and human coronavirus diseases. Nutrition, 83:111089.
Robinson PC, Richards D, Tanner HL, Feldmann M. 2020. Accumulating evidence suggests anti-TNF therapy needs to be given trial priority in COVID-19 treatment. Lancet Rheumatology, 2(11):e653-e655.
Ruan Q, Yang K, Wang W, Jiang L, Song J. 2020. Clinical predictors of mortality due to COVID-19 based on an analysis of data of 150 patients from Wuhan, China. Intensive Care Medicine, 46(5):846-848.
Sami N, Ahmad R, Fatma T. 2020. Exploring algae and cyanobacteria as a promising natural source of antiviral drug against SARS-CoV-2. Biomedical Journal, 44(1):54-62.
Saxena AK, Singh K, Su HP, Klein MM, Stowers AW, Saul AJ, Long CA, Garboczi DN. 2006. The essential mosquito-stage P25 and P28 proteins from Plasmodium form tile-like triangular prisms. Nature Structural and Molecular Biology, 13(1):90-91.
Sci-Hub | Human papillomavirus is a necessary cause of invasive cervical cancer worldwide | 10.1002/(sici)1096-9896(199909)189:1<12::aid-path431>3.0.co;2-f (no date). Available at: https://sci-hub.hkvisa.net/10.1002/(sici)1096-9896(199909)189:1%3C12::aid-path431%3E3.0.co;2-f (Accessed: 14 July 2021).
Snow RW, Guerra CA, Noor AM, Myint HY, Hay SI. 2005. The global distribution of clinical episodes of Plasmodium falciparum malaria. Nature, 434(7030):214-217.
Sobrino F, Sáiz M, Jiménez-Clavero MA, Núñez JI, Rosas MF, Baranowski E, Ley V. 2001. Foot-and-mouth disease virus: a long known virus, but a current threat. Veterinary Research, 32(1):1-30.
Soria-Guerra, R.E., Ramírez-Alonso, J.I., Ibáñez-Salazar, A. et al. 2014. Expression of an HBcAg-based antigen carrying angiotensin II in Chlamydomonas reinhardtii as a candidate hypertension vaccine. Plant Cell, Tissue and Organ Culture, 116(2):133–139.
Specht EA, Mayfield SP. 2014. Algae-based oral recombinant vaccines. Front Microbiology, 5:60.
Sun JB, Holmgren J, Czerkinsky C. 1994. Cholera toxin B subunit: an efficient transmucosal carrier-delivery system for induction of peripheral immunological tolerance. Proceedings of National Academy of Sciences, 91(23):10795-10799.
Sun M, Qian K, Su N, Chang H, Liu J, Shen G. 2003. Foot-and-mouth disease virus VP1 protein fused with cholera toxin B subunit expressed in Chlamydomonas reinhardtii chloroplast. Biotechnology Letters, 25(13):1087-1092.
Suzuki JY, Bauer CE. 1992. Light-independent chlorophyll biosynthesis: involvement of the chloroplast gene chlL (frxC). Plant Cell, 4(8):929-940.
Twyman RM, Schillberg S, Fischer R. 2005. Transgenic plants in the biopharmaceutical market. Expert Opinion on Emerging Drugs, 10(1):185-218.
Tzachor A, Rozen O, Khatib S, Jensen S, Avni D. 2021. Photosynthetically Controlled Spirulina, but Not Solar Spirulina, Inhibits TNF-α Secretion: Potential Implications for COVID-19-Related Cytokine Storm Therapy. Marine Biotechnology, 23(1):149-155.
Ulmer JB, Valley U, Rappuoli R. 2006. Vaccine manufacturing: challenges and solutions. Nature Biotechnology, 24(11):1377-1383.
Valenzuela P, Medina A, Rutter WJ, Ammerer G, Hall BD. 1982. Synthesis and assembly of hepatitis B virus surface antigen particles in yeast. Nature, 298(5872):347-350.
Walmsley AM, Arntzen CJ. 2000. Plants for delivery of edible vaccines. Current Opinion Biotechnology, 11(2):126-129.
Walboomers, J. M. M., Jacobs, M. V., Manos, M. M., Bosch, F. X., Kummer, J. A., Shah, K. V., Snijders, P. J. F., Peto, J., Meijer, C. J. L. M., & Mu�oz, N. 1999. Human papillomavirus is a necessary cause of invasive cervical cancer worldwide. In The Journal of Pathology, 189(1):12–19.
Wang J, Thorson L, Stokes RW, Santosuosso M, Huygen K, Zganiacz A, Hitt M, Xing Z. 2004. Single mucosal, but not parenteral, immunization with recombinant adenoviral-based vaccine provides potent protection from pulmonary tuberculosis. J Immunology, 173(10):6357-6365.
Yano A, Maeda F, Takekoshi M. 2004. Transgenic tobacco cells producing the human monoclonal antibody to hepatitis B virus surface antigen. Journal of Medical Virology, 73(2):208-215.
Zapanta PE, Ghorab S. 2014. Age of Bioterrorism: Are You Prepared? Review of Bioweapons and Their Clinical Presentation for Otolaryngologists. Otolaryngol Head and Neck Surgery, 151(2):208-214.